By: Jason Spruell
Polyimides as a Versatile Platform
Polyimides are an important class of polymeric materials that are used in a wide variety of applications ranging from filtration membranes and aerospace composites electronics-grade coatings and adhesives. Polyimides are known to have excellent chemical resistance, mechanical properties, and high temperature performance. For this reason, polyimides are often used in place of more conventional materials such as glass, metals, and even steel for structural components and composites. In addition, the tunability of their structures, electrical properties, and their intrinsically low coefficient of thermal expansion make polyimides ideal for use in the advanced semiconductor packaging architectures that power most of our modern consumer electronics.
Although the properties furnished by polyimides can provide superior performance in many commercially-viable applications, some of these same properties also present challenges to the manufacture and processing of polyimides themselves. To better understand these challenges, it is useful to review the traditional manufacturing methods used to produce polyimides (Figure 1). These polymers are typically formed in a two-step process through iterative condensation polymerization steps. The initial reaction between dianhydrides and diamines is an exothermic process that produces the polyamic acid intermediate. This step typically necessitates the use of polar solvents, which can be difficult to remove in downstream operations. From this polyamic acid precursor, two distinct routes may be used to synthesize the final polyimide structure: (1) thermal transformation, and (2) chemical imidization.

Figure 1. Traditional methods to generate polyimide materials and process into geometries.
Conventional Imidization Approaches have Limitations
In the thermal transformation route, the polyamic acid precursor is processed through traditional solvent processing techniques such as: film casting, solution spinning of fibers, and solution casting of membranes. The polymer geometries within these films or fibers are produced in this solution casting process, but their final properties are only defined upon a high temperature cure-in-place. This curing step drives the formation of the final polyimide structure via imide condensation and the subsequent removal of water as a byproduct. Several processing and manufacturing challenges result from the need for these high temperatures and the evolution of gaseous water in the final processing step. The high processing temperatures – typically greater than 300 °C – require the use of monomers that do not decompose in the manufacturing process. The nature of the monomers used, including the inherent rigidity and aromatic/aliphatic character can affect the cure temperature as well as the final polyimide properties. Likewise, the evolution of gaseous water during the curing of the polyimide films and fibers can lead to structural and optical defects such as pin-holes, blisters, and delaminations in the final polyimide product.
In order to overcome these thermal cure-in-place challenges, the industry has developed alternative chemical imidization processes that drive the formation of polyimides at room temperature using solution-phase dehydration agents. The most common chemical imidization method involves the use of acetic anhydride and pyridine as the chemical dehydration agents. This transformation liberates the water byproduct directly into solution, allowing the resultant polyimide to be easily filtered and subsequently processed into a desired geometry. However, despite avoiding the challenges of thermal cure-in-place by removing water prior to the final processing step, chemically imidization strategies to generate polymides can present a different set of processing challenges. For example, solutions of the resultant polyimide (if it is soluble) will have much lower absolute solubilities in typical process solvents, and also have higher viscosities than the initial polyamic acid. These higher viscosities can limit the processability of the polymer into a desired geometry. Likewise, in many cases the resultant polyimide has poor solubility, and must be melted at extremely high temperatures prior to processing into its final form. In some cases, depending on the exact structure of the polyimide, solution processing cannot be made viable nor can thermal processing be achieved without degrading the polymer itself. For these reasons, conventional chemical imidization methods are limited by the scope of diamine and dianhydride monomers used in their synthesis, requiring judicious choice of these components to be compatible with process conditions and not necessarily optimized for final application performance.
Chemical Isoimidization Technology Provides the Solution
These challenges associated with both the thermal transformation and conventional chemical imidization routes have been mitigated with a third and very useful alternative processing method. This method involves the use of a chemical isoimidation process and has started to become a preferred method for commercial-scale polyimide manufacturing. Halocarbon Electronics Solutions has worked with leaders in the industry to develop high purity chemical isoimidization agents to overcome the limitations of conventional acetic anhydride-based chemical imidization methods, while still eliminating the need for a thermal cure-in-place.
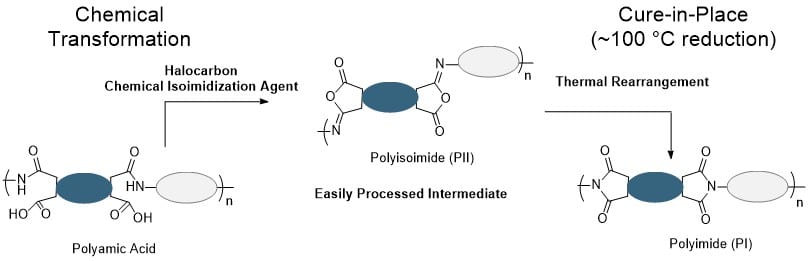
Figure 2. Polyimide synthesis via the Polyisoimide easy to process intermediate.
Halocarbon Electronics Solutions’ chemical isoimidization agents are high purity, electronics-grade, process chemicals that serve as the chemical dehydration agents. These process chemicals drive the highly selective formation of a polyisoimide intermediate. The polyisoimide is a dehydrated structure formed from the polyamic acid, and is actually an isomer of the final polyimide itself. The intrinsic properties of polyisoimides provide several advantages to both the design and processing of polyimides. For instance, as compared to the polyimide form, the isomeric polyisoimides have superior solubility in a broad range of process solvents, they also have lower intrinsic solution viscosities as well as lower melt viscosities. Each of these attributes allow for polyisoimide solutions to be prepared at higher solids concentrations, while still providing lower viscosities to enable more economical and scalable solution processing. More importantly, the Halocarbon chemical isoimidization agent is a drop-in replacement for the acetic anhydride used in conventional chemical imidization processes, thus not requiring any additional capital investments, labor requirement, or changes to existing processing equipment or infrastructure. Polyisoimides are easy to process: once a film is cast from the polyisoimide solution, their lower melt viscosity of these materials ensures that the polyisoimide film will self-level at relatively low temperatures to form more ideal films with fewer optical and structural defects such as pinholes, blisters, and disrupted polymer geometries. Because no volatiles are generated during the thermal rearrangement cure, the polyisoimides may be cured in sealed or sandwiched geometries with little fear of delamination defects. Once the ideal polyisoimide film is produced, it can be thermally transformed from the kinetically trapped polyisoimide form into the thermodynamic polyimide at relatively low temperatures (typically ~100 °C lower than direct thermal transformation temperatures). Molecular orientation can be also be enhanced by stretching the polyisoimide material during the thermal transformation thereby increasing mechanical properties and potentially introducing anisotropies.
The benefits of using the polyisoimide method:
- Lower temperature cure (typically 100 °C lower than the polyamic acid direct cure temperature)
- Increased material compatibility through the lower temperature cure (such as epoxy mold compounds)
- Reduction in defects such as pin-holes, blisters, and delaminations
- Broader toolkit of monomers through removal of processing limitations, i.e. potential to use more rigid monomers or monomers with reduced solubility
- Broader scope of process solvents
- More economical solution processing
- Reduction in cured film stress
- Ability to introduce anisotropy via directional stretching in the polyisoimide state
- Avoidance of water outgassing during the cure
- Ability to process thicker films or polyimide structures that are sandwiched within other layers
Polyisoimide routes have been considered for use in advanced applications such as electronic packaging, displays, aerospace composites and high temperature applications in the form of coatings, thin films, tapes, composites, and adhesives. However, the chemical isoimidization agents used in these processes have not always been available at scale to support commercial growth, or at the purity levels required for the end-use applications. However, as trends in the electronics industry continue to require thinner and lighter materials, the use of advanced polyimides have started to be considered in these applications. Critical to acceptable performance in many of these applications – especially for electronic applications – is the need for high quality monomers and all chemical reagents used in manufacturing. Halocarbon chemical isoimidization agents are produced in high purity and at scales to support commercial use and implementation.
In summary, polyimides are an important class of materials which, by virtue of the very properties which make them useful, suffer from processability challenges. By utilizing the polyisoimide intermediate, many of those processing challenges can be overcome while still achieving the same final polyimide structure. Moreover, this route is a drop-in to existing chemical imidization processes and existing manufacturing assets. Researchers, engineers, and OEMs in the polyimide supply chain should consider utilizing this upgraded approach to the polyimide process for improved processability, increased monomer scope, defect reduction, and lower temperature processing among others which could lead to economic efficiencies and cost reductions.
If you are a company currently producing or you are interested in polyimides or polyisoimides, we welcome your comments below. Contact us for more information or reach out directly to our sales manager.
Leave a Reply